Bioactive Compounds and Nutritional Value of Cagaita (Eugenia dysenteric) during its Physiological Development
- DOI
- 10.2991/efood.k.200729.001How to use a DOI?
- Keywords
- Native fruit; bioactive compounds; proximate composition; phenolics; antioxidants
- Abstract
The Cerrado is the second largest vegetation formation in Brazil, after the Amazon, and is the richest tropical savanna in the world in terms of biodiversity. This study aimed to evaluate cagaita fruits for bioactive compounds and nutrients during their physiological development. The fruits were assessed for phenolic compounds, antioxidant activity, vitamin C, and β-carotene in the period between 10 and 37 Days after Anthesis (DAA); the basic chemical composition and mineral levels were estimated at 18 DAA. Bioactive compounds acted mainly as a result of the defense system, especially in younger fruits, whereas the vitamin C content was relatively low throughout fruit development. The basic chemical composition of cagaita fruits was characterized by high moisture content, and lower protein, carbohydrate, and ash contents, and considerable lipids levels. Most of the physiological changes in the fruits started from the 27th day onward, which may indicate the beginning of climacteric in the fruit, culminating in the synthesis and degradation of compounds, leading to ripening. The cagaita fruit presents great potential for agricultural applications and shown potential use in the food and pharmaceutical industries.
- Graphical Abstract
- Copyright
- © 2020 International Association of Dietetic Nutrition and Safety. Publishing services by Atlantis Press International B.V.
- Open Access
- This is an open access article distributed under the CC BY-NC 4.0 license (http://creativecommons.org/licenses/by-nc/4.0/).
1. INTRODUCTION
The Cerrado is the second largest vegetation formation in Brazil, after the Amazon, and is the richest tropical savanna in the world in terms of biodiversity, concentrating one-third of the nation and 5% of the world’s flora and fauna [1]. The Cerrado flora includes several fruit trees with great potential for agricultural applications, with fruits that are traditionally consumed fresh or processed into juice, liqueurs, ice creams, jellies, and candies by the local population [2].
An increase in fruit consumption is related to their functional properties such as the presence of bioactive compounds with antioxidant activity, which may present additional physiological effects even in small quantities [3,4]. Several studies have reported the health benefits from regular consumption of fruits, vegetables, and grains containing antioxidants such as phenolic compounds, vitamin C, and carotenoids [5–7]. Phenolic compounds are important constituents of various fruits and vegetables, and the quantification of these substances reveals information about the antioxidant activity, quality of food, and potential health benefits [8]. In plants, tannins are characterized as phenolic compounds of high molecular weight that precipitate proteins, including the salivary proteins from the oral cavity. These properties are fundamental to the role of tannins in plant protection against pathogens and herbivores [9].
Carotenoids play a key role in fruits as an accessory pigment in photosynthesis, acting as a collector of energy and protecting against photo-oxidation [10]. Besides, some carotenoids are converted into vitamin A, with an important nutritional role. Other benefits include the reduction of the risk of degenerative diseases, prevention of cataract formation, reduction of aging-related macular degeneration, and reduced risk of coronary heart disease. The synthesis and accumulation of phenolic compounds, carotenoids, and vitamins, in fruits is affected by factors such as species, variety, handling, weather, ripening stage, and storage conditions [11,12]. In general, the evaluation of fruit development is based on the physical attributes associated with the changes in the chemical characteristics. Secondary metabolites, such as phenolics and oxidants also form a part of the plant composition; thus, studies on these compounds are required [13]. The study of the developmental stages of the fruits, besides being extremely important in elucidating the physiology and determining physiological compost, is also important in determining the best harvest point when it is necessary to extract a compound of interest, which may undergo oscillation in its synthesis during development.
Among the various native fruits of the Cerrado, the cagaita (Eugenia dysenteric) stands out as a yellow-colored globular fruit, containing one to three white seeds, coated with a slightly acidic pulp. Very little is known about the behavior of bioactive compounds and the most appropriate evaluation method during the developmental stages of cagaita fruits. Hence, the purpose of this study was to investigate the behavior of nutritional and bioactive compounds during the development of cagaita fruits.
2. MATERIALS AND METHODS
2.1. Sampling
The study was initially performed in a native area with a typical Cerrado vegetation, located in the city of Abadia-GO, latitude –16°45′26″ and longitude –49°26′15″. Fruits were selected at random from 30 specimens of E. dysenteric species, and marked in different plant positions, with colored woolen yarns at the time of anthesis. After formation, the fruits were collected and divided into two equal batches. At intervals of four days from anthesis, about 40 fruits were collected, packed in low-density polyethylene bags, and transported to the Food Analysis Laboratory of the School of Agronomy of the Federal University of Goias, where they were selected based on physical integrity and absence of mechanical and pathogenic damage. The experiment was conducted in a Completely Randomized Design (CRD), in a factorial treatment arrangement consisting of sampling points. Antioxidant potential, phenolic compounds, hydrolyzable tannins, vitamin C, and β-carotene levels were assessed after 10, 14, 18, 23, 27, 31, 34, and 37 days after anthesis, in four replicates. The basic chemical composition (moisture, ash, protein, lipids, carbohydrates, and minerals) was evaluated from 18 days after anthesis, up to 37 days, in triplicate.
2.2. Standards and Reagents
Ethyl ether, sodium hydroxide, ethyl alcohol, acetone, methyl alcohol, potassium persulfate, vanillin, hydrochloric acid, acetate, sulfuric acid, l-ascorbic acid, and oxalic acid were purchased from Vetec (Rio de Janeiro, Brazil). Methyl red, urea, gum Arabic, ferric ammonium sulfate, gallic acid, bromine cresol green, boric acid, potassium sulfate, and copper sulfate were purchased from Dinamica (Diadema, São Paulo). Butylhydroxytoluene (BHT), Folin–Ciocalteu reagent, 2,2-Diphenyl-1-picrylhydrazyl (DPPH), 2,2′-Azino-bis(3-ethylbenzothiazoline-6-sulfonic acid) (ABTS), and 6-hydroxy-2,5,7,8-tetramethylchroman-2-carboxylic acid were purchased from Merck (Darmstadt, Germany). Catechin, thiourea, 2,6-Dichloro-phenol-indophenol (DFI), and 2,4-Dinitrophenylhydrazine (DNPH) were purchased from Sigma-Aldrich (São Paulo, Brazil).
2.3. Preparation of Extracts
Ether, alcoholic, and aqueous extracts were prepared sequentially. 2.5 g sample was placed in a glass beaker and covered with aluminum foil to avoid contact with light, to which 50 mL ethyl ether was added and stirred with a magnetic stirrer for 1 h. The extract was filtered through a Buckner funnel with filter paper into another beaker covered with aluminum foil. After filtration, the volume was made up to 50 mL with ethyl ether. The filtered extract was placed in an amber vial and stored at −18°C. The remaining residue was dried for use in alcoholic extraction as described below.
Ethyl alcohol was added in the ratio of 1:20 into the same filter paper containing the dry residue, and the mixture stirred for 1 h to obtain an ethanolic extract. Filtration was performed with the same care provided to the ether extract. The solution was brought up to final volume with ethyl alcohol and the extract was frozen-stored in an amber vial. For aqueous extraction, distilled water at 1:20 ratio was added to the filter paper containing the dried residue and stirred for 1 h. Then, the extract was filtered and the volume was made up with distilled water. The filtered extract was placed in an amber vial and stored at −18°C.
2.4. DPPH Assay
At least three different dilutions were prepared in test tubes, in triplicate. 0.1 mL of each extract dilution was transferred to a test tube with 3.9 mL of DPPH reagent (0.06 mM DPPH) and homogenized in a shaker, taking care to maintain a dark environment. An aliquot of 750 μL BHT solution was placed in a bucket and 1.5 mL DPPH solution was added. Absorbance readings were taken at the following time: 0, 1, 2, 3, 4, 5, 10, 15, and 20 min. For the blank, 750 μL sample in 1.5 mL methanol was used. The control was prepared with 750 μL methanol in 1.5 mL DPPH. For the sample, an aliquot consisting of 750 μL and 1.5 mL DPPH was added into the cuvette, and the absorbance readings were performed at the following times: 0, 1, 2, 3, 4, 5, 10, 15, and 20 min at 517 nm in a UV Rayleigh – 1800 spectrophotometer, according to Rufino et al. [14]. The antioxidant activity was expressed according to Mensor et al. [15] as described below:
2.5. ABTS Assay
The ABTS∙+ free radical scavenging assay was performed according to Re et al. [16] in triplicate. The ABTS·+ radical is formed through a chemical reaction between ABTS solution and potassium persulfate in a 1:0.5 stoichiometric ratio, following which, the ABTS·+ solution is diluted in ethyl alcohol to obtain the absorbance measurement of 0.70 (±0.02) at 754 nm and an equilibrium temperature of 30°C. The absorbance was measured in a UV Rayleigh – 1800 spectrophotometer, at 1, 4, and 7 min after sample addition. Standard Trolox curves were used and the results were expressed as μmol TEAC per g sample (Trolox equivalent antioxidant activity) in triplicate, according to Rice-Evans et al. [17].
2.6. Total Phenolic Content
The total phenolic content was determined by the Folin–Ciocalteu method in a UV Rayleigh – 1800 spectrophotometer at 760 nm, in triplicate [18]. Briefly, 1 mL aliquots of the extracts were transferred to 10 mL flasks containing 5 mL distilled water, and 1 mL of 25% sodium carbonate, to which 1 mL Folin–Ciocalteu reagent was added, and the volume was made up with distilled water. After 30 min, the absorbance was measured at 760 nm. The phenolic compounds were quantified in each extract using external calibration curves, and the results were expressed as mg Gallic Acid Equivalent (GAE) per 100 g sample.
2.7. Vitamin C and β-Carotene
The vitamin C content was determined by the colorimetric method as described by Strohecker and Henning [19]. Vitamin C was extracted with 0.5% oxalic acid under stirring and determined in the extract after filtration, using DNPH and ascorbic acid as standard. The reading was performed in a UV Rayleigh – 1800 spectrophotometer at 520 nm, and the results were expressed as mg ascorbic acid per 100 g sample. β-carotene was extracted with acetone:hexane (4:6) and estimated by spectrophotometry according to Nagata and Yamashita [20], and the results were expressed as mg per 100 g, as follows:
2.8. Hydrolyzable and Condensed Tannins Content
The tannin content was estimated spectrophotometrically as described by Price et al. [21], with adaptations by Barcia et al. [22]. For tannin extraction, 1 g sample was mixed with 50 mL methanol under stirring for 1 h. After filtration, an aliquot of 1 mL was removed and 5 mL vanillin:HCl (1:1) (vanillin 1% in methanol, hydrochloric acid 4% in methanol) was added and allowed to react for 15 min. The absorbance was read at 500 nm in a UV Rayleigh – 1800 spectrophotometer, and the results were expressed as mg catechin per 100 g sample. Hydrolyzable tannins were estimated according to Brune et al. [23], with adaptations by Barcia et al. [22]. For the extraction of hydrolyzable tannins, 1 g sample was mixed with 50 mL methanol under stirring for 10 min and allowed to stand for 1 h. After filtration, 2 mL extract were removed and 8 mL ferric ammonium sulfate (FAS) solution (89% urea:ethyl acetate = 1:1; 10% Gum Arabic 1% in deionized water; and 1% ferric ammonium sulfate 5% in 1M hydrochloric acid) was added and allowed to react for 15 min. Readings were taken at 680 nm in a UV Rayleigh – 1800 spectrophotometer, and the results expressed as mg gallic acid per 100 g sample.
2.9. Basic Chemical Composition
Moisture, ash, protein, lipids, and carbohydrate content was determined according to the methodologies of Association of Official Analytical Chemists [24], as follows: moisture was determined by the gravimetric method in an air circulation oven at 105°C until constant weight; ash was determined by weight loss of the material after incineration in muffler at 550–600°C; protein was determined by the Kjeldahl method, based on the determination of total nitrogen, and protein content was calculated by multiplying the total nitrogen by a factor of 5.75; lipids were determined by extraction with organic solvent (ethyl ether) using the extractor device Soxhlet type; and the carbohydrate content was estimated by difference, according to the equation:
2.10. Statistical Analysis
The experiment was conducted in a CRD, and the treatments were arranged in a factorial scheme consisting of sampling points, using the Sisvar software (Lavras, Brazil). The average time intervals were subjected to regression coefficients, and the models were selected according to the significant F-test and coefficient of determination.
3. RESULTS AND DISCUSSION
3.1. Phenolic Compounds and Antioxidant Activity
Significant total phenolics levels were observed (p < 0.05) in the aqueous and ether extracts during cagaita fruit development. The phenolic content levels exhibited a double sigmoid growth behavior, starting with a high level, followed by a decrease from 18 to 27 DAA, with a further increase in the concentration and a slight decrease in the aqueous and ethanolic extracts (Figure 1). The high levels of antioxidant action in the early stages of development, especially those related to DPPH, meet the high levels of phenolics found in the same period. However, with development, there is a drop in the phenolic content, which is also reflected in the antioxidant activity. The increase in antioxidant activity at the end of development is concomitant with the increase in β-carotene and vitamin C levels during the metabolic process. According to Shahidi and Naczk [26], phenolics in plants and fruits play an important role in growth and reproduction, besides being an antipathogenic agent and contributing to pigmentation. This may explain the higher levels of phenolic compounds in the greener fruits (10 DDA), which are in the growth phase involving the synthesis of pigments such as chlorophyll. Chitarra and Chitarra [27], described that young fruits present higher phenolics concentration in tissues, once they have important functions in the plant, acting as a defense mechanism, and decreasing during development.
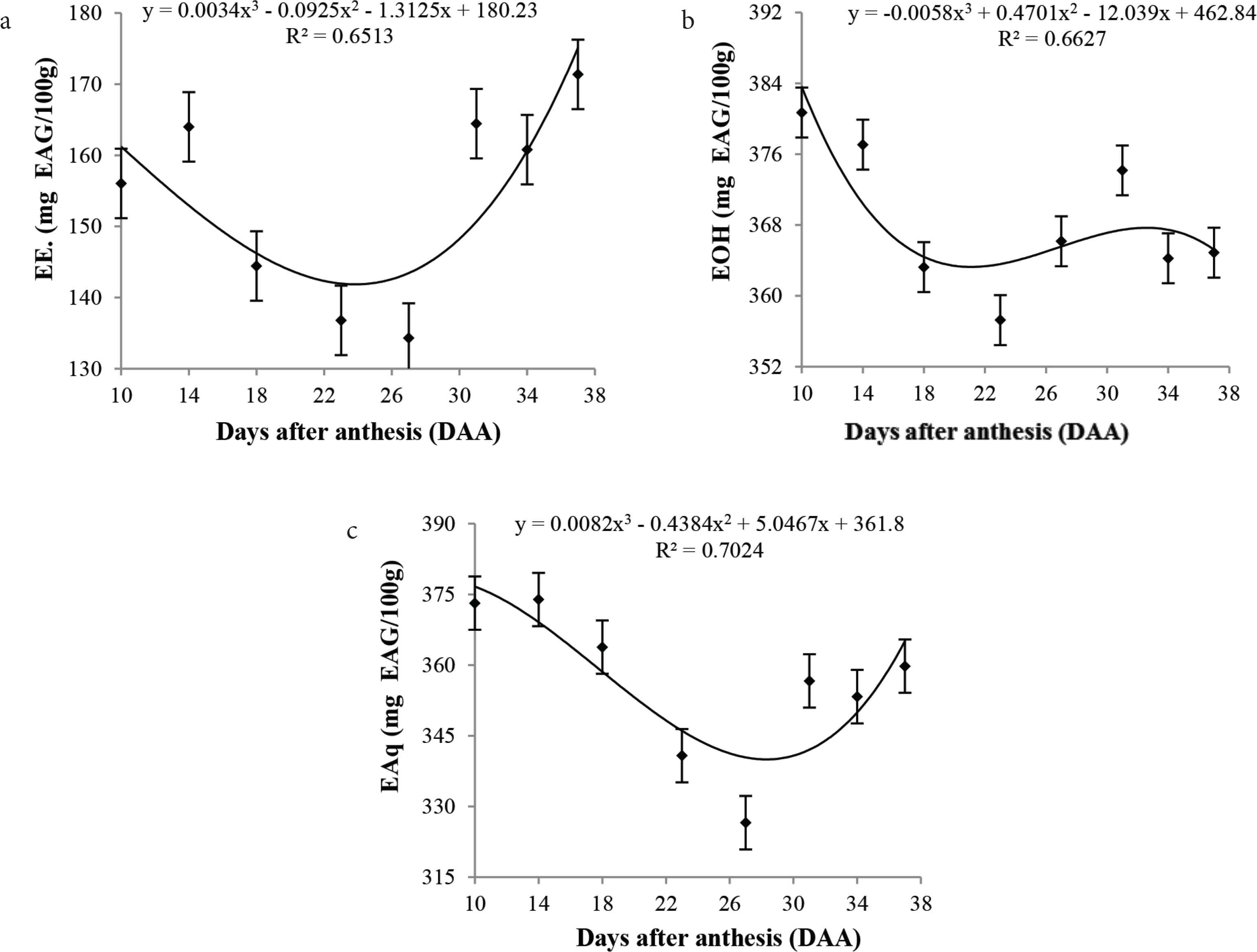
Mean values, regression equations, and determination coefficients of the phenolics values of (a) ether, (b) ethanolic, and (c) aqueous extracts during the development of cagaita (Eugenia dysenteric) fruits collected in Abadia-GO.
The best extraction was determined for the ethanolic extract, with significantly higher levels and better accuracy results when compared to other extracts. Significant results were found for the antioxidant potential of cagaita assessed by the DPPH assay (p < 0.05), showing high antioxidant capacity during fruit development. However, analysis of the curves has shown that the percentage discoloration increased at the beginning of development and decreased from 28 DAA (Figure 2). This increase is due to the intense metabolic activity of the fruit, which usually involves defense mechanisms such as biosynthesis of organic molecules, mostly as side products.
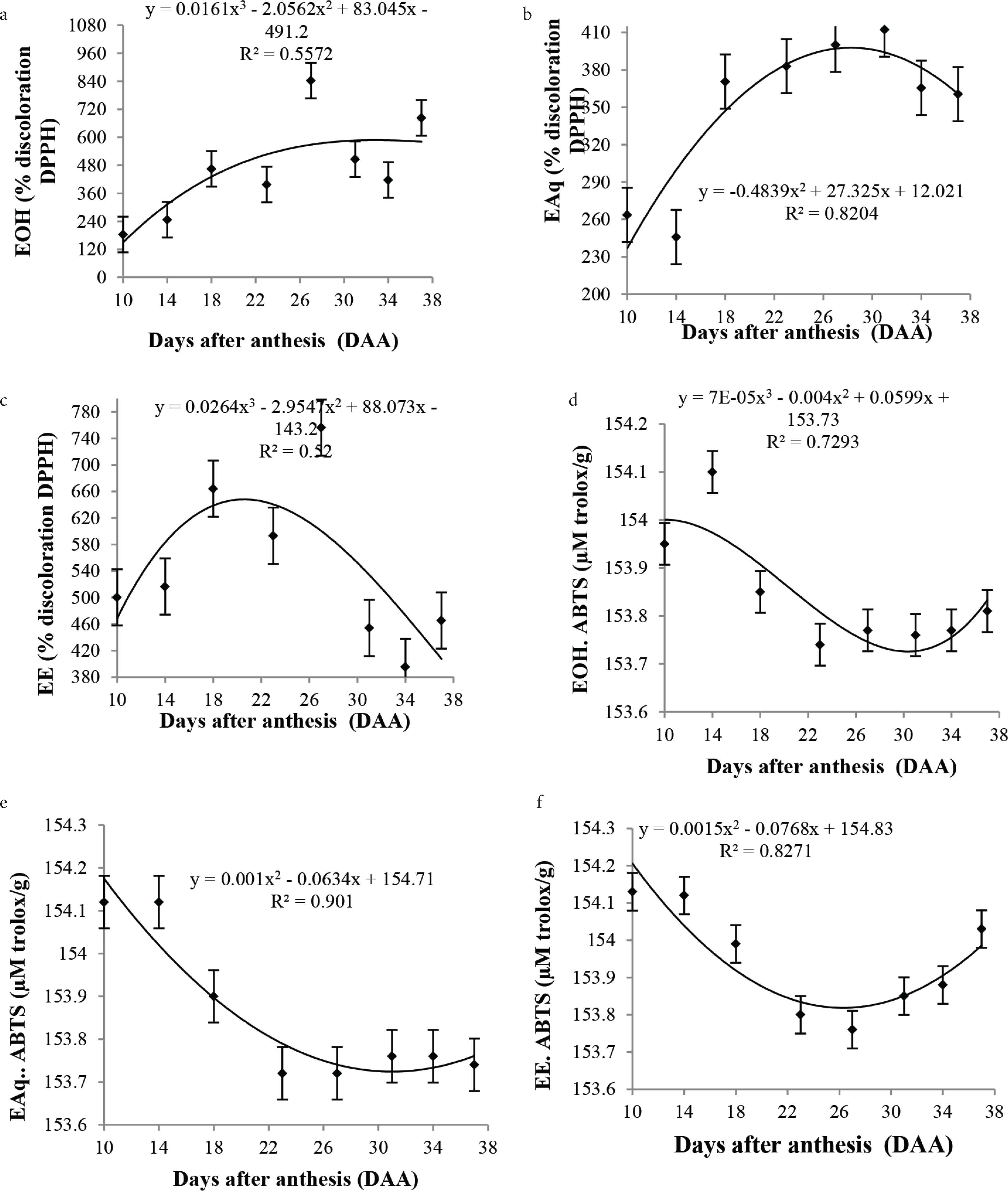
Mean values, regression equations, and determination coefficients of the antioxidant activity measured by DPPH assay in the (a) ethanolic, (b) aqueous, and (c) ether extracts, and by ABTS assay in the (d) ethanolic, (e) aqueous and (f) ether extracts during the development of cagaita (Eugenia dysenteric) fruits collected in Abadia-GO.
No significant differences were observed in the antioxidant capacity measured by the ABTS assay, indicating a low potential to capture free radicals. The ABTS values observed in all extracts during the fruit development were similar, demonstrating that this method may not be effective to evaluate the antioxidant activity of cagaita fruits (Figure 2). Due to various types of radicals and different sites of action, there is no single method capable of safely and accurately representing the true antioxidant activity of a substance [28].
3.2. Vitamin C and β-Carotene
Significant changes were noted for vitamin C and β-carotene levels. As observed in the curve behavior, the vitamin C levels decreased during the fruit development possibly due to the protective effect and antioxidant activity in younger tissues, followed by a small increase at 32 DAA (24.78 mg/100 g), and further decrease until the fruit attains full maturity (Figure 3a).
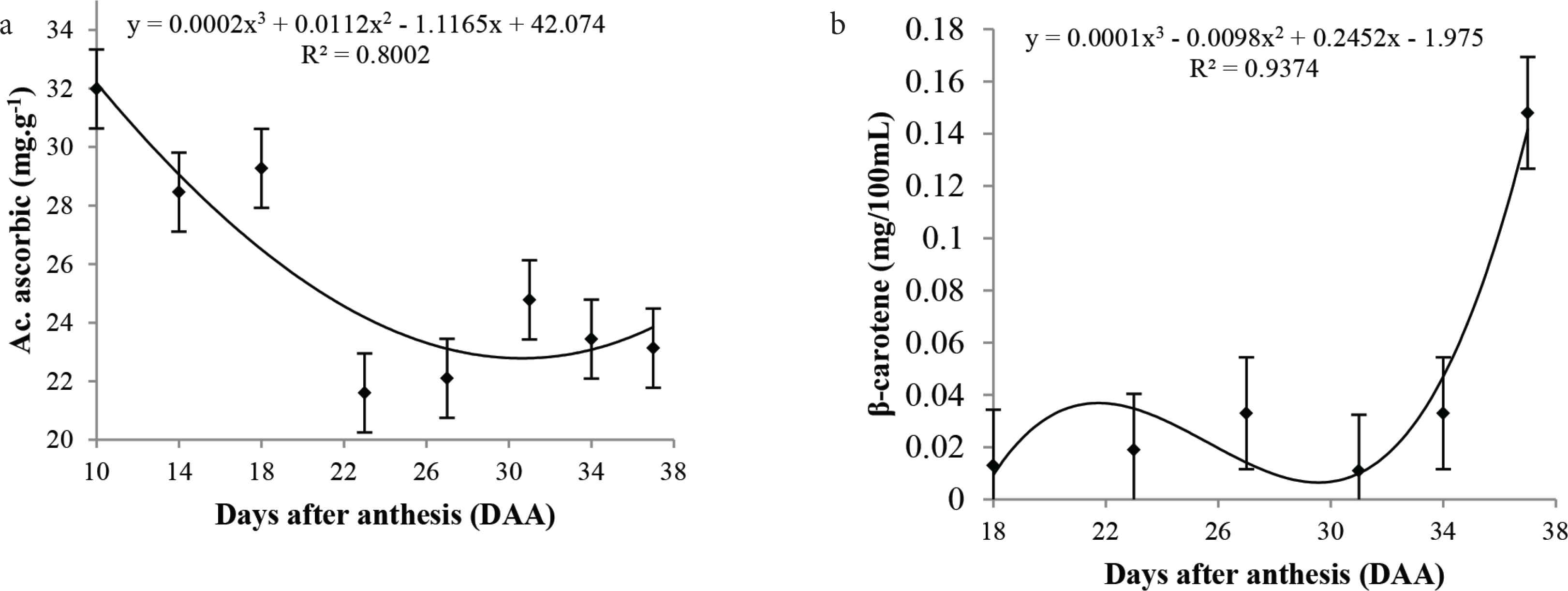
Mean values, regression equations, and determination coefficients of the (a) vitamin C and (b) β-carotene during the development of cagaita (Eugenia dysenteric) fruits collected in Abadia-GO.
β-Carotene levels remained almost constant until 34 DAA, with a fast increase from this period (Figure 3b), indicating a possible activation of the biosynthetic pathway of this pigment corresponding to fruit ripening. According to Krinsky [10], in plants, carotenoids play an important role in photosynthesis as an accessory pigment, in the collection of energy and protection against photo-oxidation. Besides, β-carotene is also a vitamin A precursor with health benefits, acting as an antioxidant, and reducing the risk of degenerative diseases [29].
3.3. Hydrolysable and Condensed Tannins
The decreased in tannin content, which possibly led to the loss of fruit astringency with ripeness, while higher levels of hydrolyzable tannins were noticed in the fruit throughout the development, especially from 10 to 18 DAA (Figure 4b). Hydrolyzable tannins are esters of glycolic acids and glycosylated ellagic acids. The hydroxyl groups of sugar are esterified with phenolic acids: gallic acid (gallotaninins) and hexahydroxydiphenic acid (ellagitannins) [30]. According to Vilas Boas et al. [31], there is polymerization of these compounds associated with fruit ripening, with a consequent decrease in astringency, sweetness, and acidity.
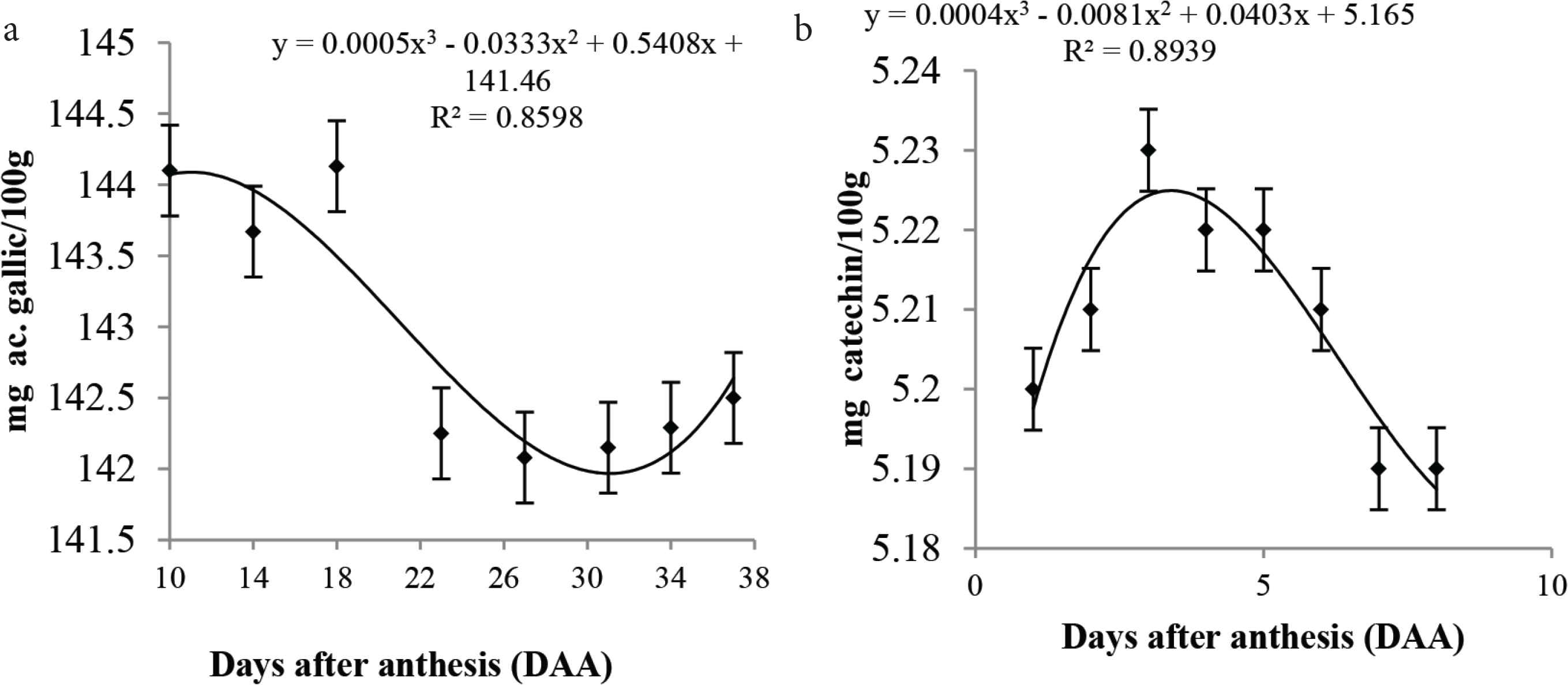
Mean values, regression equations and determination coefficients of (a) hydrolyzable tannins and (b) condensable tannins during the development of cagaita (Eugenia dysenteric) fruits collected in Abadia-GO.
Taiz and Zeiger [32], fungal invasion triggers the transcription of messenger RNA in plants and fruits, encoding phenylalanine ammonialyase thereby increasing its amount in the plant, thus stimulating the synthesis of a class of phenolic compounds, including tannins, which is also responsible for fruit astringency.
3.4. Basic Chemical Composition
A significant increase in moisture content was observed from 18 to 27 DAA, followed by a decline at 31 DAA, with 80.57 ± 2.6% moisture, which remained constant until full maturity (Figure 5a). According to the data, the fruit is made up of water, with an increase mainly from 27 DAA. As reported by Taiz and Zeiger [32], water occupies most of the cell volume, thus a cell must necessarily absorb water to increase its volume. This may explain the increase in moisture content in the younger cagaita fruits from 18 to 27 DDA, besides favoring the chemical and biochemical reactions.
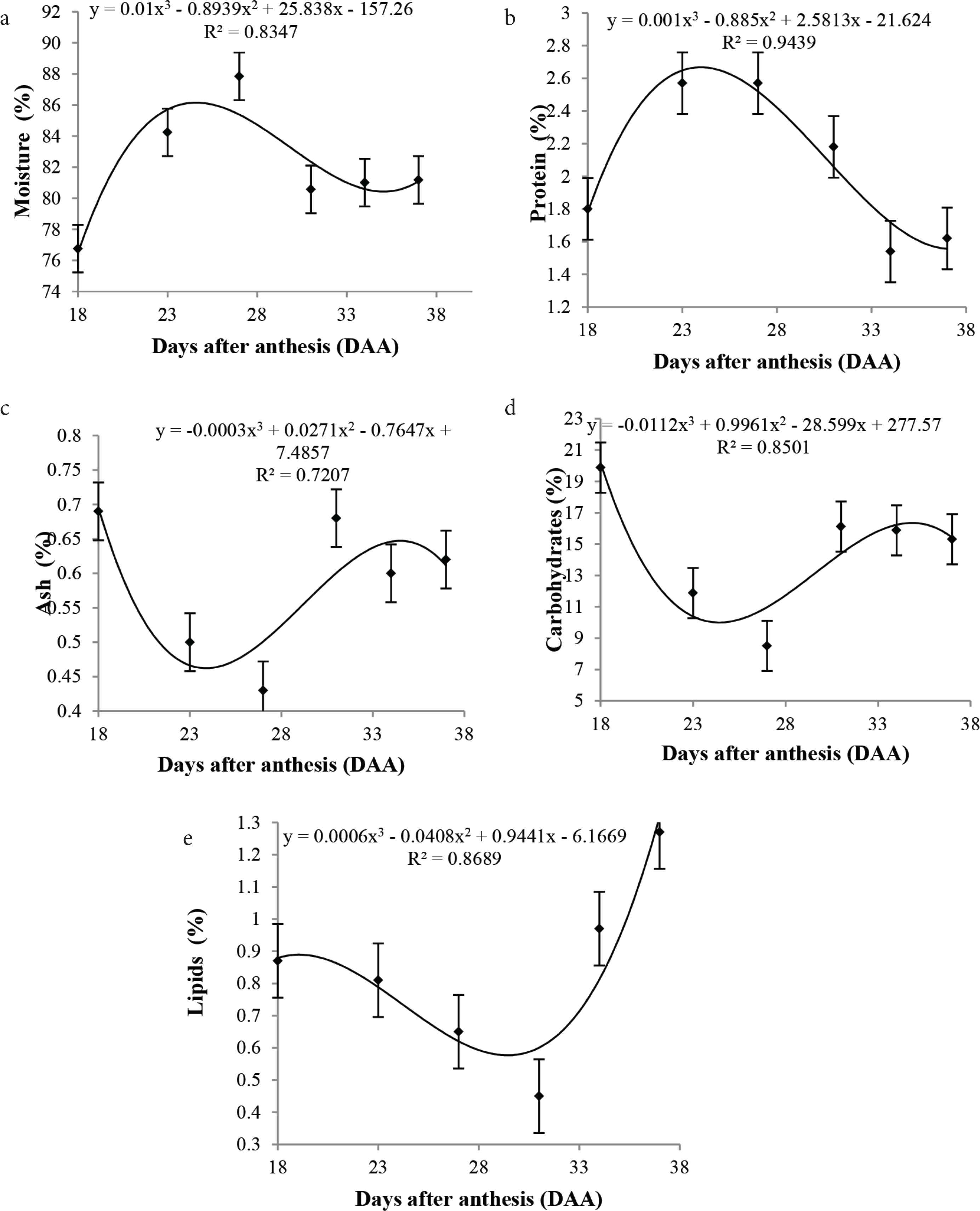
Mean values, regression equations, and determination coefficients of the basic chemical composition (a) moisture, (b) ash, (c) protein, (d) lipids, (e) carbohydrates during the development of cagaita (Eugenia dysenteric) fruits collected in Abadia-GO.
As can be seen in the curve (Figure 5b), levels of ash were affected by the moisture; a decrease in ash content was observed with increasing moisture. According to Wills et al. [33], ash content is influenced by the water content of the samples for diluting some minerals. The ash levels found in this study may have been affected by the cultivation area, since they were above the values found by other authors in mature cagaita fruits, with values of 0.18, 0.23, and 0.28 g/100 g, as reported by Cardoso et al. [34], Roesler et al. [35], and Silva et al. [2]. Ash constitutes the mineral fraction in food, consisting mainly of micronutrients, which has a direct relationship with the soil [36]. Significant changes in protein levels (p < 0.05) were observed during fruit development. There was a tendency for the protein levels to increase in the unripe fruit, corresponding to the growth stage, followed by a decrease during maturation and ripening (Figure 5c). According to Alberts [37], during the growth phase, the fruits require a programmed sequence of gene activation, i.e., the genes encode the synthesis of specific enzymes and maturation proteins, which may have caused the increase in protein levels at the beginning of fruit development. Lipid levels were also significant (p < 0.05) in response to fruit development. The lipid content decreased gradually up to 31 DAA, reaching 0.45%, possibly being used as a respiratory substrate in oxidation reactions for the biosynthesis of other molecules. However, a sudden increase was observed from 34 DAA up to 37 DAA, reaching 1.27% (Figure 5d), probably due to lowered levels of both moisture as well as the synthesis processes.
Significant differences were observed in the carbohydrate levels throughout fruit development (p < 0.05), despite the lower contents. As can be seen in Figure 5c, a decrease in carbohydrates was observed up to 27 DAA, probably due to the high respiratory rate, with higher carbohydrate consumption. From this period, an increase was observed until full maturity, reaching 15.31% at 37 DAA due to the degradation of cell wall polysaccharides.
Concerning the mineral content (Figure 6), the elements phosphorus, potassium, and magnesium were the most prevalent throughout the fruit development. Potassium showed a higher content compared to other elements, at 0.71% at 18 DAA, corresponding to fruit growth and formation. According to Laviola and Dias [38], in this stage, the plant needs a higher ratio of potassium. This nutrient plays an important role in the formation of fruit, transporting photostimulated groups in the phloem [39]. Potassium is also a nutrient required for the activation of several enzymes essential for the synthesis of organic compounds, which may explain their increase throughout the development [40]. Since the role of magnesium is mainly in the composition of the chlorophyll molecule, a lower level of magnesium is observed as the fruit matures.
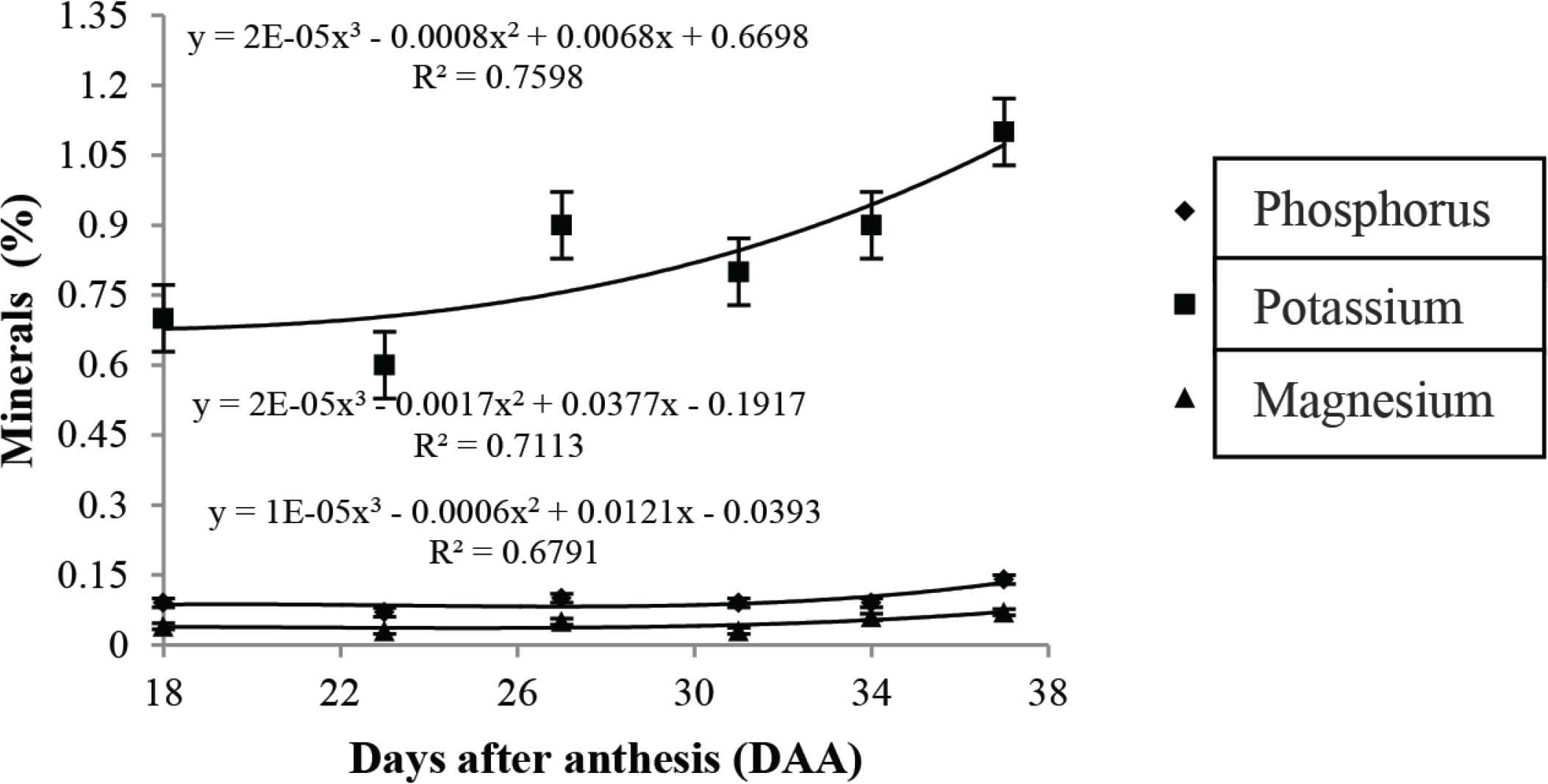
Mean values, regression equations, and determination coefficients of minerals during the development of cagaita (Eugenia dysenteric) fruits collected in Abadia-GO.
No significant differences were observed for the other minerals (sodium, boron, copper, manganese, zinc, and iron) during fruit development. Mineral contents in fruits are highly dependent on soil fertility, climatic conditions, and cultivars [41].
4. CONCLUSION
Most of the physiological changes in the fruits started from the 27th day onward, which may indicate the beginning of climacteric in the fruit, culminating in the synthesis and degradation of compounds, leading to ripening. Bioactive compounds act in defense mechanisms, especially in unripe fruits. At the end of development, the fruit showed significant amounts of β-carotene, phenolics and vitamin C respectively, which reflected directly in the antioxidant percentages. The basic chemical composition changed throughout the development of cagaita fruits, with significant changes from 27 DAA, except for lipid levels.
CONFLICTS OF INTEREST
The authors declare they have no conflicts of interest.
AUTHORS’ CONTRIBUTION
MM, LG, EP and CD contributed to designed and performed experiments, data collected, and analyzed, a manuscript written. ER and EV contributed to the data collected and analyzed. AP, JB, EP and CD contributed to analyzed data, manuscript critical evaluation. EP and CD contributed in conceptualized and designed the experiment; analyzed and critical evaluation of data.
ACKNOWLEDGMENTS
The authors are grateful for the financial support provided by
Footnotes
REFERENCES
Cite this article
TY - JOUR AU - Monik Maryelle Moreira da Silva AU - Edson Pablo Silva AU - Lismaíra Gonçalves Caixeta Garcia AU - Eduardo Ramirez Asquieri AU - Eduardo Valério de Barros Vilas Boas AU - Aline Priscilla Gomes da Silva AU - Jianbo Xiao AU - Clarissa Damiani PY - 2020 DA - 2020/08/10 TI - Bioactive Compounds and Nutritional Value of Cagaita (Eugenia dysenteric) during its Physiological Development JO - eFood SP - 288 EP - 297 VL - 1 IS - 4 SN - 2666-3066 UR - https://doi.org/10.2991/efood.k.200729.001 DO - 10.2991/efood.k.200729.001 ID - daSilva2020 ER -