miRNAs as Regulators of Antidiabetic Effects of Fucoidans
- DOI
- 10.2991/efood.k.190822.001How to use a DOI?
- Keywords
- Diabetes mellitus; miRNAs; fucoidan; antidiabetic effects; sulfated polysaccharides
- Abstract
Diabetes mellitus is a metabolic disease with a high mortality rate worldwide. MicroRNAs (miRNAs), and other small noncoding RNAs, serve as endogenous gene regulators through binding to specific sequences in RNA and modifying gene expression toward up- or down-regulation. miRNAs have become compelling therapeutic targets and play crucial roles in regulating the process of insulin resistance. Fucoidan has shown potential function as an α-amylase inhibitor, which may be beneficial in the management of type 2 diabetes mellitus. In recent years, many studies on fucoidan focused on the decrease in blood glucose levels caused by ingesting low-glucose food or glucose-lowering components. However, the importance of miRNAs as regulators of antidiabetic effects was rarely recognized. Hence, this review emphasizes the antidiabetic mechanisms of fucoidan through regulation of miRNAs. Fucoidan exerts a vital antidiabetic effect by regulation of miRNA expression and thus provides a novel biological target for future research.
- Copyright
- © 2019 International Association of Dietetic Nutrition and Safety. Publishing services by Atlantis Press International B.V.
- Open Access
- This is an open access article distributed under the CC BY-NC 4.0 license (http://creativecommons.org/licenses/by-nc/4.0/).
1. INTRODUCTION
Diabetes is one of the most common metabolic disorders in the world and the prevalence of diabetes has been increasing in the last 50 years [1,2]. As of 2013, there were 382 million people with diabetes worldwide, and this number is expected to rise to 592 million by 2035 [3]. Type 2 diabetes mellitus (T2DM), named non-insulin-dependent diabetes mellitus, is a disease characterized by insulin resistance (IR) and impaired pancreatic β-cell function that affects more than 170 million people worldwide [4,5]. Sustained hyperglycemia is the main diagnostic trait of this disease. People with T2DM are at increased risk for serious health problems, including cardiovascular disease, premature death, blindness, kidney failure, amputations, fractures, frailty, depression, and cognitive decline [6]. The mortality rate is almost double that of persons without the disorder [7]. T2DM needs to be recognized as a serious problem, and research into the best ways to prevent or treat this disorder needs urgent attention.
MicroRNAs (miRNAs) are a class of noncoding RNAs about 22 nucleotides in length [8]. They play crucial roles in regulation of developmental processes, cell differentiation, cell proliferation, and apoptosis pathways through interference with gene translation by sequence-specific binding to protein-coding mRNAs [9,10]. In recent years, miRNAs have been widely used in therapeutics of complex diseases researches, such as diabetes. Glycans were confirmed to affect the levels of target miRNAs, which offered the new strategies and approaches to deal with the challenge of T2DM.
Fucoidans consist of a series of sulfated fucose-rich polysaccharides that are mainly derived from various species of brown seaweed [11]. It is a type of complex and heterogeneous sulfated polysaccharide, consisting of
2. TYPE 2 DIABETES MELLITUS
The pathogenesis involves abnormalities in insulin action, insulin secretion, and endogenous glucose output. IR represents the key role in the pathogenesis of T2DM [15]. It causes multiple deleterious effects to contribute to diabetes-related complications and is defined as the impaired sensitivity of tissues, such as liver, skeletal muscle, and adipose tissues. Up till now, the major mechanisms of inducing IR by destroying the insulin signaling cascade have been identified.
Fucoidan was regarded as a crucial glucose regulator that could act on genes and enzymes existing on mitochondria, nucleus, endoplasmic reticulum (ER), and cell membrane. A fucoidan-blocked mitochondrial pathway was discovered on a T2DM or inflammatory cells, on which fucoidan were found to be able to decrease the level of tumor necrosis factor-α (TNF-α) and Bid, which were crucial factors to promote mitochondrial effete pathways. The activated TNF-α would trigger the activity of c-Jun N-terminal protein kinase and caspase. The Bid promote the Bax concentrate on the membrane of mitochondria in the form of homodimer to change the mitochondrial membrane. Thus, the cytochrome c, a key gene determining the β-cell apoptosis, was delivered out of the mitochondria to injure the β-cell via facilitating the activity of caspases 3 and 9 [16]. What’s more, the fucoidan could restore the insulin synthesis of β-cell via Sirt-1-dependent signaling pathway. It could promote the glucagon-like peptide-1 (GLP-1) and GLP-1 receptor combining on the surface of β-cell to ameliorate its death. Then, the phosphorylation of GAPDH was activated by the combination to elevate the Sirt-1 activity. Sirt-1 plays a crucial role in insulin secretion, which can promote the activity of phosphorylation of protein kinase B (AKT) and forkhead box protein A2 (FOXA2). AKT could inhibit the phosphorylation of FOX O1 and pancreatic and duodenal homeobox-1 (PDX-1), thus together with FOXA2 activating PDX-1 to promote insulin production [17]. The adenosine monophosphate-activated protein kinase (AMPK) pathway induced by ER stress could be ameliorated by fucoidan, thus maintain glucose homeostasis. It is reported that fucoidan could promote the activity of liver kinase B1 and AKT, which could activate the phosphorylation of downstream substance AKT, which control the glucose uptake and metabolism by activating AMPK. Then the silence of mechanistic target of rapamycin complex 1 (mTORC1) (a tumor suppressor) caused by AMPK could increase the insulin receptor substance (IRS) level to promote glucose metabolism [18]. In addition, fucoidan could stimulate insulin release from β-cell through cyclic AMP (cAMP) pathway. It was found that fucoidan could act on the upstream substance of cAMP, the activation of adenylyl cyclase, and the silence of phosphodiesterase would control the breakdown of cAMP. cAMP plays a vital role in controlling the release of insulin through regulating the concentration of Ca2+ on both sides of cell membrane (Figure 1).
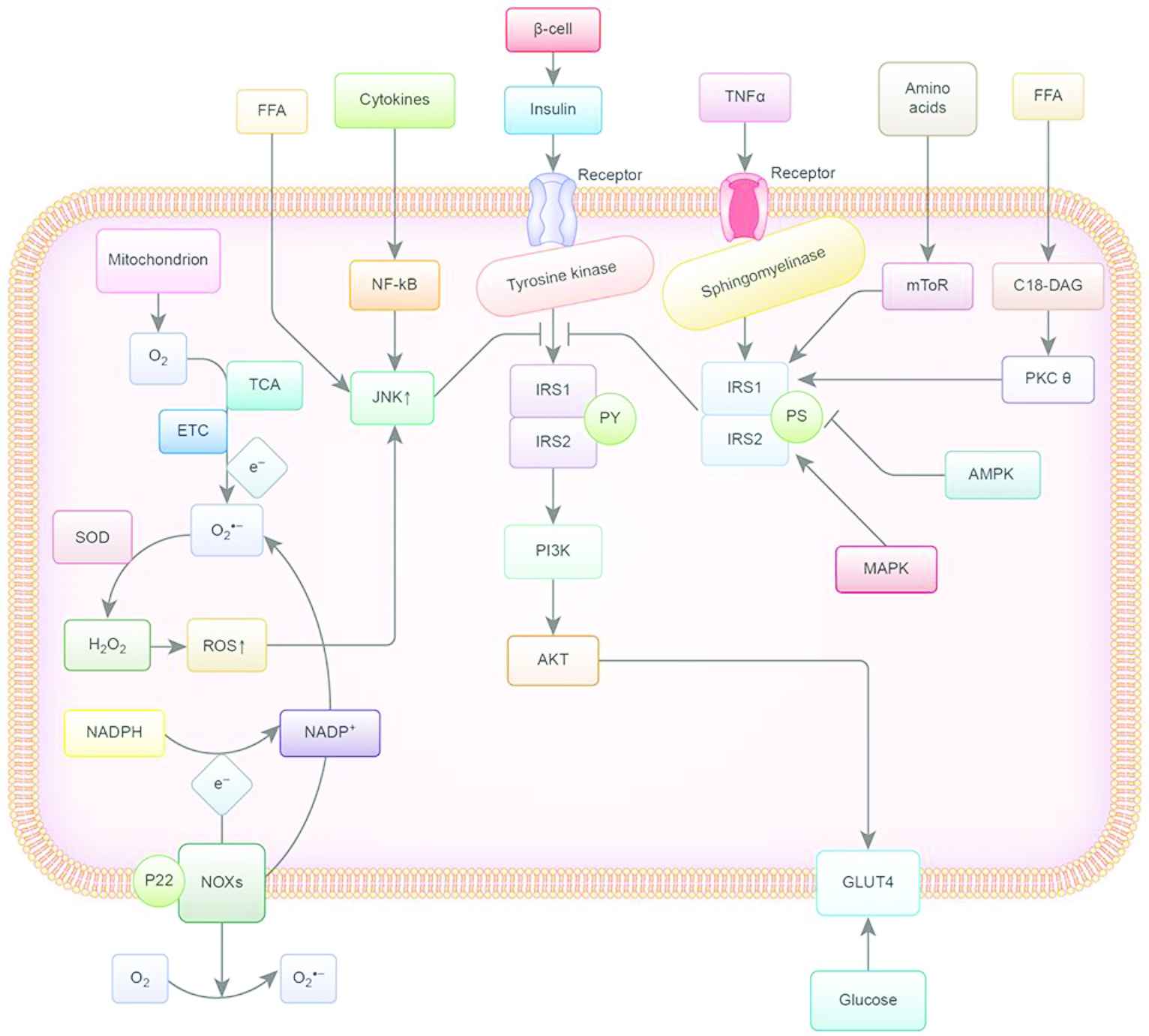
This flow chart summarizes the mechanisms of IR. Y, tyrosine; S, serine; IRS-1/2, substrate 1/2 of the insulin receptor; PI3K, phosphoinositide kinase 3; TNF-α, tumor necrosis factor-α; GLUT4, glucose transporter 4; JNK, c-Jun-N terminal kinase pathway; FFA, free fatty acids; ROS, Reactive oxygen species; NF-κB, nuclear factor kappa-light-chain enhancer of activated B cells; AKT, phosphorylation of protein kinase B; TCA, tricarboxylic acid cycle; ETC, electron transport chain; SOD, superoxide dismutase enzymes; P22, membrane partner protein; NOXs, NADPH oxidases; MAPK, mitogen-activated protein kinase; mToR, mechanistic target of rapamycin complex; PKC-θ, protein kinase C theta; AMPK, Adenosine monophosphate activated protein kinase.
Insulin is secreted by the β-cells, stimulating the tyrosine kinase activity and subsequently phosphorylating various intracellular substrates, including IRS-1 and IRS-2. Phosphorylated IRS-1 and IRS-2 trigger the insulin signal via activation of phosphatidylinositol-4,5-bisphosphate 3-kinase-protein kinase B (PI3K-AKT). There are several pathways that can induce IR [19]. First, excessive availability of lipids causes translocation of protein kinase C-θ (PKC-θ), and high concentrations of amino acids cause activation of the rapamycin-sensitive mTOR pathway, thus inducing serine phosphorylation of IRS-1. Besides, TNF-α changes the insulin receptor signaling pathways by diverting IRS-1 away via sphingomyelinases. The JNKs, which are activated by circulating cytokines and free fatty acids, can also directly inhibit phosphorylation of IRS-1 and can contribute to chronic IR. Reactive oxygen species (ROS) are by-products of oxygen consumption and cellular metabolism, which are formed by partial reduction of molecular oxygen. Nicotinamide adenine dinucleotide phosphate (NADPH) oxidases and mitochondria are two major contributors of ROS. They have been associated with IR and directly interfere with insulin signaling. Hyperglycemia, the hallmark metabolic abnormality associated with T2DM, is the key point in the treatment of diabetes. Recently, therapies directed at other coincident features, such as obesity and IR, have also been a major focus of research and therapy [20]. Food and the functional components rich in phytochemicals, such as polyphenols, polysaccharides, oligosaccharides, and so on, can be very effective antidiabetic agents [15,21–25].
3. FUCOIDANS
Fucoidans are commonly sulfated marine polysaccharides and have wide spectrum of bioactivities [11,22]. They are constituents of several species of brown seaweed and some marine invertebrates (such as sea urchins and cucumbers). Fucoidans were first isolated by Kylin [26] from brown algae: Ascophyllum nodosum, Fucus vesiculosus, Laminaria digitata, and Laminaria saccharina. Since then, fucoidans have been identified in more than 70 species of brown algae (Phaeophyceae), in the body wall of some marine invertebrates such as sea cucumber (Holothuroidae), and in the egg jelly coat of sea urchins (Echinoidea) [27]. Ecklonia cava, F. vesiculosus, and Cladosiphon okamuranus were most widely studied species for fucoidans [28]. Polysaccharides have distinct functional properties that are influenced by their structures. Fucoidans are a group of fucans, i.e., sulfated polysaccharides extracted from brown seaweeds and are characterized by fucose-rich sulfated groups. Other examples of fucans are ascophyllans (xylofucoglycuronan and xylofucomanuronan) and sargassans (glycuronofucogalactan). The position of sulfate groups has great influence on the beneficial biological activities of marine sulfated polysaccharides. The bioactive properties of fucoidans extracted from different species of seaweed depend on their compositional structure, charge density, distribution, and bonding of the sulfate substitutions [29]. Most of fucoidans have complex chemical compositions. Except for fucose and sulfate, they contain monosaccharide-, glucuronic acid-, and acetyl-groups, which can make structural analysis quite complex. Fucoidans extracted from different species of brown seaweed and by different methods showed a great variety in structures. Ponce et al. [30] found that fucoidan extracted at room temperature was composed mainly of
In the previous few decades, the biological activities of fucoidans isolated from different marine species have been widely reported (Figure 2). They include antivirus, antitumor, immunomodulatory, anti-inflammatory, blood lipids reducing, anticoagulant, antithrombotic, antioxidant, and anti-complementary properties; also, hepatoprotective, uroprotective, and renoprotective properties have been recorded [36]. Compared with other polysaccharides, algal sulfated fucoidans are widely available from a variety of low-cost sources and have been investigated in recent years to develop the medical drugs or functional foods.

Biological effects of fucoidans. The letters represent related polysaccharides, which had biological activities.
4. FUCOIDANS AS ANTIDIABETIC AGENTS
Fucoidans isolated from brown seaweeds had potential beneficial effects on diabetes. Moreover, different fucoidan demonstrated hypoglycemic effects and showed some variety in their mechanisms of action (Table 1). Fucoidans and their biological activities have been extensively studied and reported in the past 100 years. The excitement lies in the reports on success in the treatment of diabetes. Fucoidans were mainly used to treat diabetes-related complications, such as diabetic nephropathy and retinopathy. The major signaling pathways have been investigated and reported on to explain the mechanisms of antidiabetic effects of fucoidans (Figure 3).
Sources | Structures | Mechanisms | References |
---|---|---|---|
Undaria pinnatifida | Improve insulin sensitivity | Kim et al. [37] | |
Acaudina molpadioides |
|
Blocked mitochondrial pathway in pancreatic islet cell apoptosis via regulation of inflammatory cytokines | Wang et al. [16] |
Ascophyllum nodosum | α-(1-3) linked fucose with a low proportion of α-(1-4) linked fucose or a repeating α-(1-3) and α-(1-4)-linkage | Inhibit α-amylase activity | Kim et al. [38] |
Turbinaria ornata | Presence of alkane proton in two methyl group, alkyl at sulfonyl attached proton, methoxy attached proton at H-4, present 3-linked |
Inhibit α-amylase activity | Lakshmanasenthil et al. [39] |
Sargassum wightii | Presence of alkane proton in two methyl group, alkyl at sulfonyl attached proton, methoxy attached proton at H-4, present 3-linked |
Inhibit α- |
Kumar et al. [40] |
The trials of fucoidans linked to diabetes mellitus
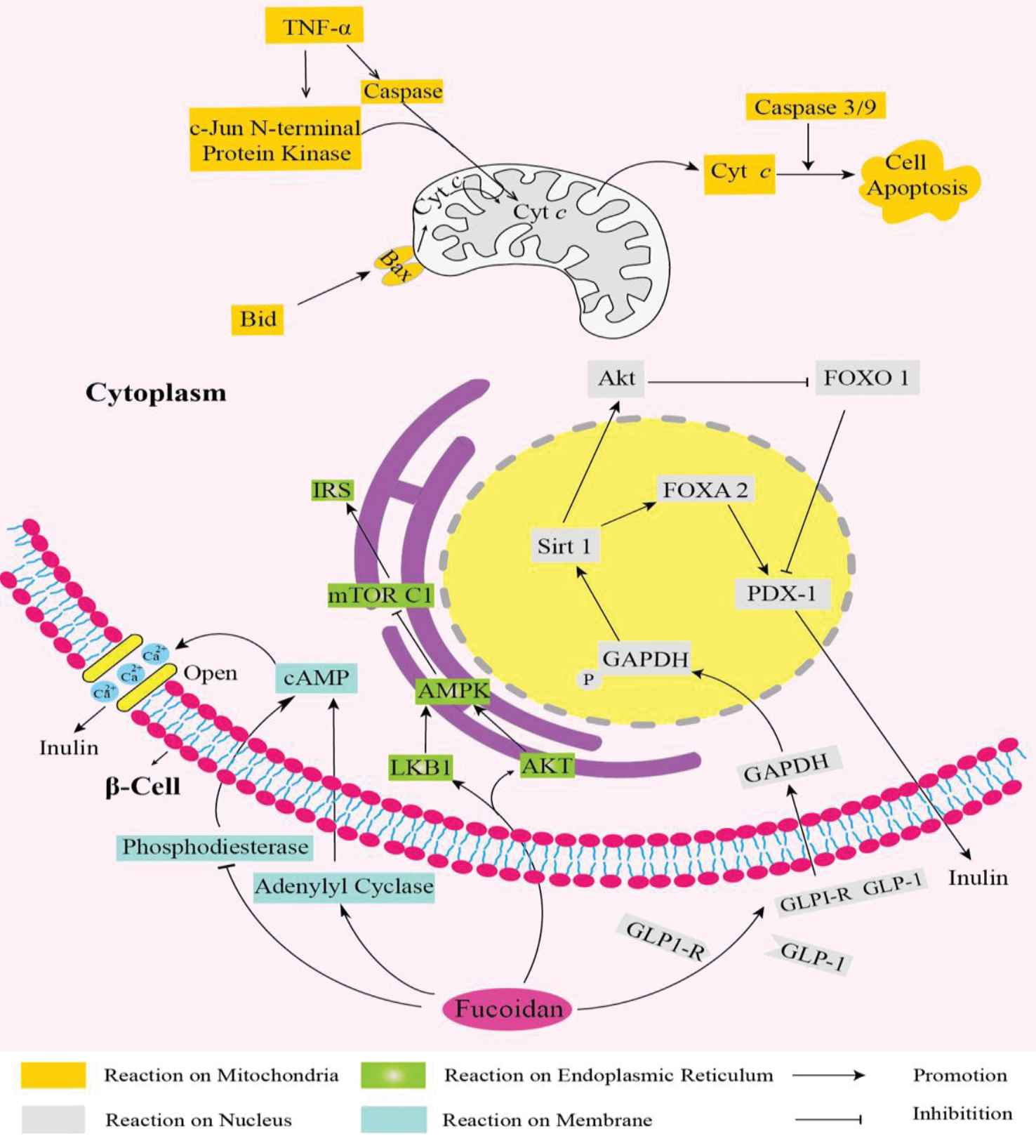
Main signaling pathways recruited to adjust the blood glucose.
The phenomenon has been confirmed in a range of in vitro and in vivo trials. Studies showed that fucoidans could act as α-glucosidase inhibitors in vitro, which was associated with diabetes. What is more, the inhibitory effect of fucoidan on α-glucosidase was largely dependent on its structure. Moreover, the structural characteristics of fucoidan were linked to the inhibition of α-amylase. Compared with the fraction from F. vesiculosus, fucoidans from A. nodosum with their high sulfate and medium-molecular weight exhibited stronger α-amylase inhibitory activity (Kim et al., 2015).
Fucoidan from F. vesiculosus decreased the fasting blood glucose and the weight of diabetic mice. Using nuclear magnetic resonance (NMR) spectroscopy, it was found that the major components were the α-l-Fuc,
5. MicroRNA AND T2DM
miRNAs are synthesized by RNA polymerase II and form transcripts that fold back on themselves to form short hairpins. RNA polymerase II transcribes the long RNA precursors (pre-miRNAs) [46], processed by RNase III enzymes, such as Drosha and Dicer [47,48], and then incorporated into the RNA interference effector complex (RISCs) [49], which mediates the degradation of targeted mRNA transcripts and translational arrest, and negatively regulates gene expression at the posttranscriptional level by binding to the 3′-untranslated region of target RNAs [50,51] (Figure 4). Since the discovery of miRNAs in Caenorhabditis elegans, more than 28,600 miRNAs have been found in plants, animals, and viruses [52]. About one-third of known miRNAs were embedded within introns of protein-coding genes. They are co-transcribed with their host genes, which make that miRNA and protein expression are regulated in a coordinated fashion [53]. From studies of miRNAs functions in cancer cells, many of the functional roles have shown the involvement of miRNAs in human diseases [46]. Almost 60% of human protein-coding genes were potentially regulated by miRNAs in health and disease [54]. Mechanisms involving miRNAs in disease processes are very complex; some diseases were even associated with more than 20 miRNAs. Currently, with the development of investigation, an increasing number of researchers have focused on the relationship between diabetes and miRNAs.

A schematic diagram of miRNA synthesis. RNA polymerase II transcribes the long RNA precursors (pri-miRNAs) and processed by Drosha and Dicer and incorporated into the RNA interference effector complex, which mediate degradation of targeted mRNA transcripts and translational arrest, negatively regulate gene expression at the posttranscriptional level by binding to the 3′-untranslated region of target RNAs.
There is no doubt that miRNAs will directly or indirectly affect our health. Abnormal expression of miRNAs was observed in plasma, serum, and whole blood in patients with T2DM [55–58]. By using microarray screening and miRNA network inference, target miRNAs, i.e., miR-15a, miR-20b, miR-21, miR-24, miR-126, miR-191, miR-197, miR-223, miR320, and miR-486 levels were lowered, while miR-28-3p expression was increased in plasma of diabetic patients. When levels of serum miRNA in newly diagnosed patients with T2DM and prediabetes or susceptible individuals were measured, serum levels of miR-9, miR-29a, miR-30d, miR34a, miR-124a, miR-146a, and miR-375 levels in T2DM patients were significantly higher than those in susceptable people. When compared with prediabetic individuals, patients with T2DM had serum levels of miR-9, miR-29a, miR-34a, miR-146a, and miR-375, which were significantly upregulated in T2DM patients. However, there were no significant differences in the expression of miRNAs between prediabetic patients and susceptible individuals [56]. There have also been reports that abnormal expression of miRNAs was found in the whole blood and exosome of the metabolic syndrome population [55]. Among patients with T2DM, miR-27a, miR150, miR-192, miR-320a, and miR-375 were upregulated, and then the increase levels of miR-27a and miR-320a were associated with higher FBG. Moreover, obesity also could influence the expression of miRNAs in patients with diabetes. Meanwhile, aberrant patterns of expression of miRNAs were observed in adipocytes [59,60].
MiRNAs play a key role in the pancreas [61,62], where they regulate insulin production and insulin secretion in β-cells of the pancreatic islets [63,64]. MiR-375 was important for normal pancreatic islet formation and had many effects on islet β-cells, including insulin expression and secretion, islet β-cell proliferation. MiR-375 directly reduced PDK-1 protein levels, which in turn led to a decreased glucose stimulation that otherwise would have initiated expression of the insulin gene and DNA synthesis in β-cells [65].
Consistent with the above evidence, the overexpression of miR-30d inhibited the insulin gene expression, which was related to the deficiency of insulin synthesis in diabetic patients [66]. The early inflammatory factors of diabetes mellitus type 1 interfere with insulin secretion and destroy islet β-cells. This process coincides with abnormal expression levels of miR-21, miR-34a, and miR-146 [67–69]. Meanwhile, the activation of p53 pathway induced by palmitic acid (PA) was shown to lead to the up-regulation of miR-34a and miR-146 levels, resulting in the pancreatic β-cell apoptosis and the decrease of nutrient-induced insulin secretion [70–73].
More recently, elegant investigations have validated the hypothesis that miRNAs are implicated in the target tissue for insulin. Herrera et al. [63] reported that the presence of 283 DMT2-related miRNAs in adipose, liver, and muscle tissues by comparing microarrays from hyperglycemic, intermediate glycemic, and normoglycemic rats. They identified 29 miRNAs that showed significant differences, nine in adipose tissue, 18 in liver, and two in muscle. The expression patterns of five miRNAs were associated with the strain-specific glycemic phenotype. MiR-222/miR-27a and miR-195/miR-103 were upregulated in adipose tissue and liver, respectively, while miR-10b was downregulated in muscle. Likewise, He et al. [74] suggested that three paralogs of the miR-29 family were upregulated in muscle, fat tissue, and liver of diabetic rats. Overexpression of the miR-29 family in 3T3-L1 adipocytes reduced the glucose uptake of insulin stimulation. Compared with non-insulin-stimulated, it was found that miR-29a and miR-29c were highly expressed in insulin sensitive tissues [75,76]. Taken together, these reports have revealed the crucial roles of miRNAs in the etiology of type 2 diabetes.
6. miRNA-TARGETED MODULATION BY FUCOIDANS
Dietary phytochemicals have been used to change metabolism and alter diseases progression and clinical outcomes [77]. Polysaccharides or oligosaccharides from marine seaweed or resources are known to affect the levels of miRNAs. Alginate oligosaccharide or fucoidan can regulate the levels of miR-29b, miR-29c, and miR-17-5p [78–80]. In subjects with metabolic syndrome, specifically obesity, prediabetes, and T2DM, a large amount of miRNAs are deregulated, e.g. miR-21, miR-24.1, miR-27a, miR-28-3p, miR-29b, miR-30d, miR-34a, miR-93, miR-126, miR-146a, miR-148, miR-150, miR-155, and miR-223. These miRNAs significantly changed across the diabetes spectrum and were associated with measures of pancreatic islet β-cell function and glycemic control [81]. The miR-29 family has emerged as important regulators of glucose metabolism. It was among the most abundantly expressed miRNAs in the pancreas and liver and showed strong regulatory functions in obesity and diabetes [76]. The miR-29 family of miRNAs in humans comprises three mature members, miR-29a, miR-29b, and miR-29c. MiR-29 has been shown to affect glucose metabolism, lipid metabolism, and insulin responsiveness in skeletal muscle, while both miR-29a and miR-29c could regulate glucose uptake and insulin-stimulated glucose metabolism [82]. Chen et al. [83] have reported that miR-29b inhibits progressive renal inflammation and fibrosis in type 2 diabetes in db/db mice. Under diabetic conditions, miR-29b was largely downregulated in response to advanced glycation end product. Besides, Zhu et al. [84] have revealed 32 miRNAs that were differentially expressed in gestational diabetes mellitus, including five upregulated miRNAs (hsa-miR-16-5p, hsa-miR-17-5p, hsa-miR-19a-3p, hsa-miR-19b-3p, and hsa-miR-20a-5p). These miRNAs may serve as noninvasive biomarkers. Moreover, reduction of miR-17-5p and upregulation of miR-29b-3p directly regulated circadian gene expression in the maturing islet cells of 10-day-old rats [85].
Until now, many studies have focused on miRNA-targeted modulation of fucoidan against human diseases. Wu et al. [78] showed that fucoidan inhibited breast cancer progression by dual regulation of the miR-29c/ADAM-12 and miR-17-5p/PTEN axes. Furthermore, fucoidan has been confirmed to markedly upregulate miR-29b in human hepatocellular carcinoma cells to regulate the DNA methyltransferase 3B-metastasis suppressor 1 (DNMT3B-MTSS1) axis and inhibit epithelial-mesenchymal transition (EMT) (increased E-cadherin and decreased N-cadherin). DNMT3B is an important downstream target of miR-29b. Induction of miR-29b results in suppression of DNMT3B and a consequent increase in MTSS1, which is usually repressed in human hepatocellular carcinoma. It was a novel target of DNMT3B [79]. Based on the reported studies, it was confirmed that fucoidan could upregulate the level of miR-29b and miR-29c and downregulate the level of miR-17-5p, which would contribute to treatment of type 2 diabetes. At present, only limited data are available on the role of novel antidiabetic polysaccharides or oligosaccharides from algae as miRNAs regulators. This promising field should be explored further, and the fucoidan-miRNAs relationship needs to be worked out in more detail.
7. CONCLUSION
Fucoidan has been widely used in clinical studies and is a novel therapeutic agent with potential for the treatment of diabetes. The limitation of fucoidan was their poor bioavailability. Even though the discovery in this field is still at the infancy stage, the application of fucoidan is likely to have a bright future. There is no doubt that the regulation of miRNAs by fucoidan could create novel therapeutic strategies for hypoglycemic treatment in T2DM. However, there are still some questions that need to be addressed. Due to the lack of sufficient understanding of regulation of miRNAs by fucoidan, the related research was even more difficult. Systemic approaches toward the fucoidan on antidiabetes are almost absent. It is necessary to get more studies to focus on the antidiabetic effects of fucoidan through miRNA regulation.
CONFLICTS OF INTEREST
The authors declare they have no conflicts of interest.
AUTHOR CONTRIBUTIONS
C.Z., S.-S.L., D.-S.W. and D.L. contributed in writing—original draft preparation, X.-B.Z., A.I. and H.-E.S. contributed in writing—review and editing, J.-B.X. contributed in supervision. C.Z. contributed in funding acquisition.
ACKNOWLEDGMENTS
This work was financially supported by Project of
Footnotes
REFERENCES
Cite this article
TY - JOUR AU - Chao Zhao AU - Shanshan Lai AU - Desheng Wu AU - Dan Liu AU - Xiaobo Zou AU - Amin Ismail AU - Hesham El-Seedi AU - Randolph R.J. Arroo AU - Jianbo Xiao PY - 2019 DA - 2019/09/09 TI - miRNAs as Regulators of Antidiabetic Effects of Fucoidans JO - eFood SP - 2 EP - 11 VL - 1 IS - 1 SN - 2666-3066 UR - https://doi.org/10.2991/efood.k.190822.001 DO - 10.2991/efood.k.190822.001 ID - Zhao2019 ER -